Tumour pH - Functional Aspects
SK Gupta, R Kaul
Department of Radiotherapy, Institute Rotary Cancer Hospital, All India Institute of Medical Sciences, New Delhi-110 029, India
Abstract
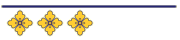
Determination of whether the pH gradient of cancer cells is lower or higher than normal is important with regard to pH regulation and to any treatment concept based on pH gradient. This article explores how an understanding of pH and tumour metabolism affect therapeutic modalities.
Introduction
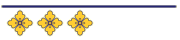
According to classical radiation biology, the radiosensitivity of tumours is mainly determined by 4 'Rs': repair, repopulation, reoxygenation, and redistribution in the cell cycle.1 The molecular events linked to these mechanisms are being investigated, together with the molecular targets that can be influenced with the aim of modulating tumour cell radiosensitivity. At the molecular level, radiation causes formation of free radicals. These radicals interact with DNA and macro-molecules in the cytoplasm membrane, causing signal transduction and gene expression. In addition to intrinsic genetically determined resistance, physiological properties primarily caused by an inadequate and non-uniform vascular network affect the responsiveness of tumours to various treatment modalities such as chemotherapy and radiotherapy. Factors which define the metabolic microenvironment encompass circulatory parameters, blood supply, transvascular and interstitial transport, and tumour pH. It is obvious that the system of the solid tumour represents a structure in which interdependence exists between different levels of molecular, cellular, histo- morphological, and physiological levels.2
Since oncogene expression may alter the metabolism of tumours, it is important to understand the relationship between tumour metabolism and pH and how it differs from that of normal tissue. This will help to manipulate gene expression in living cells. Whether the pH gradient of cancer cells is lower or higher than in normal cells is important with regard to mechanisms of pH regulation and tumour life and will affect strategies for therapeutic approaches.
Warburg showed that tumour cells undergo more aerobic glycolysis than healthy cells, changing glucose to lactic acid in abundance.3 For decades after Warburg's work, tumour pH has been taken to be an acidic medium. Until recently, pH was measured by insertion of microelectrodes directly into the tumour and was generally found to be 5.6 to 7.6 (normal tissue pH is 6.8). These values validated the research showing that tumours grow in an acidic environment. However, these measurements, which include an unknown component from damaged cells and blood released from ruptured microvessels, are now acknowledged to reflect pH in normal tissues, which also form large amounts of lactic acid although, unlike in a tumour, this is rapidly removed.
The story of tumour pH has recently evolved.4 Techniques measuring pH without the invasiveness of microelectrodes, most notably P31 magnetic resonance spectroscopy (MRS), have now become available. In 1981, the first MRS was performed in animals5 and, in 1984, it was trialled in human tumours.6 P31 MRS has confirmed that the tumour pH is neutral (7.0). In tumours, the microenvironment is rich in inorganic phosphate and large extracellular volume so the pH could not be automatically assumed for tumours. However, it has now been confirmed in several studies that pH MRS represents the pH of the tissue measured.7 This means that the pH gradient across the plasma membrane of tumours presented as pH1 > phe is the reverse of normal tissue (pH1 < phe). Since H+ and lactate move together on the monocarboxylate carrier, the distribution of H+ and lactate across the plasma membrane tends to assume the relationship (H+i) (lactate-i)/He T lactatee- = 1.
In normal tissues, pH is < pHe so lactate-i/lactate- e < 1. In solid tumours the situation is reversed and pHi is higher than pHe and lactate-i /lactate-e > 1. This means lactic acid is extruded from tumour cells and is proportional to the difference between pH1 and pHe; assuming that the pHi remains the same while pHe becomes acid, lactate will accumulate in cells.8 The correlation between high lactate levels and the incidence of metastasis in human cervical cancer have been noted. Tumours consist of normal host cells, stroma, macrophages, and tumour cells, which often occupy less than half of the volume of a tumour. One percent to 10% is contributed by blood vessels and 60% by a collagen rich matrix.9 Potentiometric methods represent H+ activity based on insertion of a pH sensitive electrode into solid tumour. P31 MRS is safer and non-invasive. Other techniques available for use are fluorescent pH indicators such as umbelliferme, fluorescence radio imaging microscopy, sampling of interstitial fluid, and use of an MRS visible extracellular marker such as 3 aminopropyl, phosphate, F19-labelled dyes, and fluoroscent dyes such as SNARF-1.10 All of these methods are currently suitable for measuring pH in experimental conditions. However, pH can also be calculated by using the Henderson-Hasselbach equation, which is only for experimental use at this time.
Effect of pH on Oncology Therapies
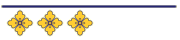
The mechanism of action of certain anticancer drugs depends on the effect of pHi and PHe. pHe affects active transport and diffusion of drugs into tumour and normal cells. The mechanism conjugates non-toxic prodrugs which release free or cytotoxic drugs at an acidic pH and releases them into the extracellular fluid. Manipulation of pH enhances the effectiveness of anti-cancer drugs. Blood flow modifiers such as flavone acetic acid increase tumour acidity and increase the cytotoxicity of chemotherapeutic drugs.11 The fact that the pH gradient of tumour cells is the reverse of that of normal tissues, an inadequate blood supply, impaired Kreb's cycle, and glycolysis are all key factors in maintaining the pH of the tumour. The change in gradient of H+ causes changes in equilibrium and energy. So, the pH of the tumor is important diagnostically as well as therapeutically. Micromilieu play an important role in the behaviour of the tumour, its progression, and the impact on therapeutic modalities. But this field requires more research to understand the tumour microenvironment, the effect on clinical oncology, and therapeutic strategies.
References
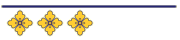
1. Hall EJ. Radiobiology for the radiologist. Philadelphia: Lippincott; 1999.
2. Hallanan DE. Tumour pH introduction. Semin Radiat Oncol 1996;6:243-244.
3. Warburg O. The metabolism of tumour. Vol II. London: Constable; 1930.
4. Vaupel P, Kallinowski F, Okunieff P. Blood flow, oxygen and nutrient and metabolic microenvironment and human tumour. Cancer Res 1989;49:6449-6465.
5. Griffiths JR, Stevens AN, Iles RA, et al. 31P-NMR investigation of solid tumours in the living rat. Biosci Rep 1981;1:319-325.
6. Griffiths JR, Cady E, Edwards RH, et al. 31P- NMR Studies of a human tumour in situ. Lancet 1983;1:1435-1436.
7. Stubbs M, Bhujwalla BM, Tozer GM. A assessment of p31 MRS as measuring pH of rat tumour and effect. NMR Biomed 1992;5: 351-359.
8. Albers C, Van Den Kerkhoff W, Vaupel P. Effect of CO2 on the pH of tumor cell present in malingant ascitic fluid. Respir Physiol 1981;45:273-285.
9. Braunschweiger PG, Schiffer RM. Effect of dexamethasone on vascular function of RIF-1 tumor. Cancer Res 1986;46:3299-3303.
10. Martinez-Zaguilan R, Martinez GM, Lattanzio F, Gillies RJ. Simultaneous measurement of intracellular pH and Ca2+ using the fluorescence of SNARF-1 and fura-2. Am J Physiol 1991;260:297-307.
11. Parkins CS, Chadwick J, Chaplin DJ. Enhancement of chlorambucil cytotoxicity by combination with flavone acetic acid in murine tumours and malignancy. Cancer Res 1993; 13:1437.
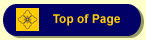
|